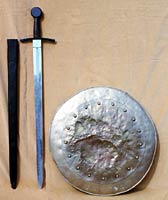
Artisans are metal
Wandering the aisles of the recent Materials Solutions trade show in St. Louis, one phrase comes to mind: old economy. Materials is an exotic field these days, but this is not the place to check out fringe semiconductors, zirconium ceramics, or the synthetic polymers that make up our increasingly Mars-ready fashions. This place is about steel, that ubiquitous but unsexy stuff that continues to form the backbone of skyscrapers, vehicles, and millions and millions of tools and machines.
The expo is sponsored by ASM International, a trade group founded by Detroit blacksmith Bill Woodside in 1913, a time when the ancient art of metallurgy had already metamorphosed into the core technology of the industrial age. The details of steelmaking have changed since then, but today’s concern is essentially the same: heat treatment. After all, you can’t make steel at room temperature. You need to get your iron glowing with heat, infuse it with carbon, and then quench the resulting matter in some sort of cooling liquid. Other substances may be added to the mix, but heat is the heart of the matter, and steel manufacturers are always on the lookout for greater control over the process. Though there are some new tools on the block – digital temperature regulators, electron microscopes for analyzing unwanted microfractures – the world of steel remains a conservative commodity market, devoid of flash or fancy. To an outsider, the booths at the Materials Solutions show quickly blur into an endless procession of valve trains, high-temp thermometers, and notices for the Thermal Spray Society.
Then there’s the booth for QuesTek Innovations. Alongside the glossy logo, against a black cloth, hang two bloodthirsty blades: a 7th-century Viking broadsword and a foot-long Danish knife called a seax – the sort of weapon that the legendary Geat hero Beowulf used to dispatch his final foe. The seax is clamped onto a chem-lab ring stand, poised above a third blade: a modern Japanese hunting knife that has a sharp, quarter-inch chunk cut out of its edge. The eyes of many a bored salesman light up at the sight of this cutlery.
The broadsword and the seax are replicas of medieval originals. Chinese hands fabricated the broadsword, which Greg Olson, the blond, 53-year-old Northwestern University professor who serves as QuesTek’s chief science officer, bought online for a couple hundred bucks. But Olson enlisted Richard Furrer, an old-school bladesmith in Sturgeon Bay, Wisconsin, to forge the seax, and Furrer didn’t skimp on authenticity. Olson points out that the handle is made of oosic, which is a polite way to say mineralized walrus penis: “Apparently, it doesn’t get slippery when it’s covered with blood.”
Walrus penis is intriguing enough, but it’s the seax blade that makes Olson grin. The material is Ferrium C69, the hardest of three fancy steel alloys that QuesTek has brought to market. Costlier than most other steels (it runs roughly $10 per pound), C69 is a breakthrough material, offering up to 50 percent greater hardness than rival alloys with similar carbon content.
But what really sets QuesTek’s materials apart is their genesis. Unlike the vast majority of new steels, which are developed by cooking up prototype materials and testing them repeatedly in the lab, QuesTek’s steels were designed entirely on computers. Employing what he calls a systems design approach, Olson and his students at Northwestern use sophisticated thermodynamic models of metal transformations to mix and match the alloys and heat treatments they need to produce the strength, hardness, and flexibility they’re looking for.
“The whole strategy is to get there by design,” Olson says. “Instead of experimenting until you find something, you completely create it beforehand at the workstation. Then you test it and see if it’s true. So far, our models have proven true.”
Though manufacturers are increasingly turning to computer models to select the best materials for each job, QuesTek’s digital quest for elite steels remains pretty radical. The ultimate goal is aerospace, where the company believes its steels can cut the weight of helicopter engine gears in half. But given the enormous amount of testing involved in building machines that carry human beings around, QuesTek will prime the pump on easier markets like collector knives, golf clubs, skate blades, and parts for the tool and die industry. More than 30 manufacturers are testing QuesTek’s alloys, but no one has signed on the dotted line just yet. Full of technological promise but short on black ink, QuesTek resembles a dotcom startup in an overwhelmingly brick-and-mortar business.
QuesTek’s business model helps explain its presence at the Materials Solutions show, but it doesn’t explain the Viking broadsword. As it turns out, the Nordic blade is just a stand-in for a mightier sword to come, because this year Greg Olson and QuesTek are planning to use their Ferrium C69 to forge the hardest sword in history.
The Japanese hunting knife on display got the gash in its cutting edge from a Ferrium C69 knife fabricated last spring by a group of students in Olson’s Engineering, Design, and Communication course. In contrast to the seax, which was forged with a hammer and anvil, the Ferrium C69 blade was machine-cut, then “case hardened,” which involved returning the piece to high temperature after it was shaped to diffuse more carbon into its outer surface. This carburization process gave the knife a superhard edge while keeping its core soft enough to prevent brittleness, the Achilles’ heel of hard steels.
After quenching the C69 blade in oil and tempering it again at 900 degrees Fahrenheit, the students clamped their blade and the Japanese knife to a Sintech testing machine, their two edges facing each other. Slowly, the machine pressed the knives together. Although the C69 blade chipped slightly, it proved victorious, slicing a quarter inch into the Japanese knife.
Olson had his students go to all this trouble because he wants to prove that his sword could, in theory, chop through a Japanese katana blade. Katanas were magnificent medieval weapons used by samurais; today, they are invested with great, almost mystical value by the collectors to whom Olson will market his broadsword. That’s partly why the students chose a Japanese hunting knife for the demo. The blade – made by MAC Corporation of Seki, Japan, a region famous for its katanas – also had the right muscle: On the Rockwell hardness scale used by metallurgists to test their wares, it ranked just shy of the C60 rating for katanas. In contrast, the carburized tip of the C69 blade was, as you might guess, nearly C69.
Olson is confident that his QuesTek broadsword will be able to cut into a samurai blade. But the professor wants more from his weapon: He wants to make a blade that could – hypothetically, of course – destroy one of the greatest supernatural beasts in Western lore. He wants to forge a Dragonslayer.
***
Cultures all over the world carry dragons in their myths – in China, the bearded, snaky monsters are even said to bring good fortune. But when you think of dragons and swords, you envision blond warriors battling foul, fire-breathing lizards over hoards of treasure. The time is usually the so-called Dark Ages, when Germanic tribes cavorted around Europe after the collapse of the Roman Empire. When their bards weren’t chanting legends of Sigurd the Dragonslayer, these tribes produced some of Europe’s first dependably powerful swords. Before then, iron blades were so flimsy that they often had to be bent back to shape in mid-battle. In addition to gaining more control over carbon infusion and quenching, the Germanic smiths perfected pattern welding – a process of folding and twisting different steels together that not only purifies and strengthens the metal but also graces the blade with gorgeous, snaky patterns that resemble monochromatic marble paper.
After his students conducted surveys of bladesmiths and sword collectors to help him determine the final design of the Dragonslayer, Olson settled on a pattern-welded, double-edged early medieval broadsword with a one-handed grip. To nail down the performance specs, Olson enlisted Michael Drout, then a doctoral student in medieval literature at Loyola University. Focusing mostly on the Völsung saga (a source of Wagner’s Ring cycle) and the Beowulf legend, Drout figured that, in addition to the phenomenal strength necessary to penetrate a dragon’s thick hide, the blade would need to have a high thermal tolerance in order to withstand fiery breath. And dragon blood is intensely acidic, which means that you’d want the corrosion resistance offered by high-chromium steel. Surveys of sword collectors also indicated that the juju (and auction price) of the blade would soar if at least some of the base iron in the alloy came from meteorites, the “sky metal” that supposedly lent King Arthur’s sword Excalibur its magical properties.
Olson aims to finish the Dragonslayer by this summer and auction it off in the fall to bring attention to his work. Given the sword’s unique history and powers, Olson hopes it will earn at least its weight – no more than 3 pounds – in gold. “We’ll donate the proceeds to the appropriate charity,” he says. “Like a university steel research group.”
Regardless of its final auction price, the sword will generate some buzz for QuesTek. The company has already applied to trademark the Dragonslayer name for a potential line of cutlery, and CEOs may one day pack Dragonslayer drivers on the golf course. But for Olson, the Dragonslayer project goes far beyond building a brand. The sword speaks to his own quest, both philosophical and practical, to transform matter into materials that people truly value. And he’s read enough history and literature to know that the deepest value of materials is not simply economic, but also aesthetic – even, in the case of meteoric iron, mystical. With his dragonslaying cybersteel, Olson hopes to engineer nothing less than a “spiritual material.”
***
The first thing Greg Olson remembers drawing when he was a kid was the atomic mushroom cloud – the ultimate symbol of scientific power in the 1950s. At his Long Island grade school, he could hardly wait until the hour when the “science cart” arrived, with its seductive beakers and Bunsen burners. In high school, he collected fluorescent crystals, set up a chemistry lab in his basement, and constructed model rockets and other excuses for setting off explosives. When he discovered that you could dig a musty chunk of rock out of the earth and turn it into metal, he was amazed. Olson asked his chemistry teacher if he could get class credit for doing the operation himself, but the man turned him down. “That’s engineering,” he sneered.
This disdain didn’t stop Olson from building a blast furnace in his driveway – made from a 5-gallon drum lined with bricks and fed by a vacuum cleaner set to blow in reverse. After firing the thing up using charcoal briquettes, he melted down old TV antennas and poured the molten metal onto the gravel driveway. But his teacher’s comment stayed with him, underscoring the fact that science and technology are two different beasts. As Olson explains today, “Science takes nature apart in order to generate theories and information. Technology puts things together to change the world.”
Olson came of age at a time when pure science – especially physics – was more highly regarded than engineering, particularly in universities. But in Olson’s field, hands-on manipulation has long been favored over, say, the analysis of chemical elements. Quoting a passage from theOdyssey, Olson points out that humans have known for thousands of years how to toughen iron by plunging it into a cooling liquid. But scientists didn’t figure out why this method worked until the late 19th century, when a German microscopist named Adolf Martens realized that what set strong and weak steel apart was not so much their chemical composition but what came to be called martensite – the dense, crazy-quilt pattern of microcrystalline structures that can form in steel during rapid quenching.
Olson studied martensite at MIT, where he analyzed the processes behind its fantastic web of imperfections, microfaults, and crystalline structures. Like H2O, which can form ice, water, and vapor, metals are not just slabs of uniform chemical bits but dynamic systems that pass through various phase transitions. Heat up iron to a sufficient degree and you get crystalline grains that easily accept the carbon atoms necessary to make steel. Cool this stuff fast enough and these grains rapidly re-form into new crystal structures that shear and smash together as if they’ve been hit by a thousand hammers. Paradoxically, these defects and deformations strengthen the overall material. Olson compares the formation of martensite to an automobile pile-up, and it can happen so fast and furiously that it generates an audible click.
Olson entered MIT a budding technologist, but while earning his PhD he became more of a scientist, doing pure research and writing papers. Olson recognized the power of science’s quest for value-free facts, but he now thinks that objective knowledge must be tempered with emotional and intuitive ways of approaching the world. “The goal of science is to be like a Vulcan and suppress your humanity,” he says. “The way we do scientific observation is reptilian. A reptile can observe you and eat you without experiencing any emotion. We are training scientists to behave that way. And we’re damaging them. When it’s taken too far, I think science does brain damage.”
Olson is serious about the neurology of the matter. “The mammalian brain, compared to the reptilian brain, is structured for emotion. Our higher-level skills of synthesis and evaluation are controlled by the limbic system, where our emotions are seated. It’s where the power is. Call it spirituality, call it what you want, but we have this awesome power that exists only in mammals.”
By the early 1980s, Olson was tired of the reptile game. Around that time, a team of metallurgists at the Royal Institute of Technology in Stockholm released the first version of a computer program called Thermo-Calc. Based on computational tools originally developed in the 1960s, the Swedish program calculated thermodynamic phase diagrams for crystalline solids such as metals and ceramics. It dawned on Olson that you could build on these tools in order to craft materials from the get-go, conforming them to performance parameters established beforehand. You could enter into the spirit of design and become an architect of metals instead of a gambler rolling the dice on your latest alloy. In other words, you could use computers to transform the reptilian science of materials into the mammalian creation of value. With this vision in mind, Olson founded the Steel Research Group, an ambitious consortium that eventually drew in university, corporate, and government players.
Olson studied during the tenure of Cyril Smith, the great MIT philosopher-metallurgist, who approached materials as intensely dynamic, complex systems whose properties – desirable and not – arise from the structures that form and interact across different scales. For the SRG, this meant developing thermodynamic computer models that could simulate these scales, from the relatively large world of 100 microns, where liquid metal forms solid crystals, down to the nanometer level, where the 3-nanometer carbide particles that give Ferrium C69 its Superman strength are born. Olson’s team even applied computational quantum mechanical techniques developed at Northwestern in order to calculate and control the subatomic interactions between the tiny crystal grains that can embrittle metals.
Orchestrating this dynamic dance was a job for a designer, not a Vulcan. Though Olson never had any formal design training, he drew inspiration from music composition. In high school, he wrote pieces for orchestra and jazz band, and during his time in Cambridge he sat in on advanced composition classes at the Berklee College of Music across the river. One course focused on Duke Ellington; at the end of the semester, Olson composed “The Loch Ness Boogie,” a piece based on Ellington’s “Swamp Fire.”
“When you compose music, you are doing a dynamic, multilevel theoretical design,” he says. “Of all forms of music, jazz is the most like materials.” In other words, metals aren’t like Vivaldi concertos, with their perfectly resolved patterns of harmony. Instead, good metals swing, embracing noise and mess along the way. “The key to materials is the control of the imperfections you need. And no form of music has as dense a defect density built into it as the structure of jazz.”
Jazzy or not, Olson’s plans were pooh-poohed by most materials scientists, who believed that the dynamics of steel were too complex and nonlinear to design. But soon after Olson took the SRG from MIT to Northwestern, his research started to bear fruit. In 1989, he and a crew of undergraduates won an award from NASA for their computer-aided design of a stainless steel alloy for the bearings in the space shuttle’s main engine turbopumps. (NASA has since defaulted to a more common, commercially available steel.) At the same time, Olson’s group attracted the attention of the Newman-Haas racing team, whose members were intrigued by the possibility of high-quality gear steels that wouldn’t strip in the course of a race.
Inevitably, Olson started to look beyond the university. “We started out with the question: Is design possible in steelmaking? When we found out the answer was yes, then the next question was: How would you get this out into the world?”
The obvious way was to license the technology from the university and start a company. Olson hooked up with Charlie Kuehmann, a student who had worked with him on the NASA project. In 1996, they founded QuesTek as a design and consulting firm. They worked with the Howmet Corporation to craft alloys for turbine engine blades and helped USG develop computational approaches to better plaster. But their focus shifted gradually to selling their steels directly to manufacturers. Though they failed to entice VCs or form partnerships with existing specialized steel companies, they scraped together $1.5 million through angel financing to help them crack this new market.
QuesTek has yet to ship production quantities of its metals, and will have to move beyond computers to real-world trials if it is to succeed. “The proof of the pudding is in component testing,” says George Krauss, professor emeritus at the Colorado School of Mines and a steel industry expert who greatly admires Olson’s work with the SRG. “You can’t just take a computer and make a new steel and say this is going to work.” Krauss also points out that great performance doesn’t get you very far if production costs are too high. QuesTek’s alloys are larded with pricey elements like cobalt and chromium, and most big steel consumers just don’t want to hear about it.
Nonetheless, Olson is confident that the high quality of QuesTek’s cybersteels will ultimately get the company into aerospace, where the big bucks are found. But in the meantime, the existing materials need to get to market.
Inside QuesTek, Olson is the big-picture guy. He is the first to admit that he remains more of a professor than a businessman: “If I were on my own, I’d blow it.” Even when it comes to something as prosaic as the possibility of Dragonslayer golf clubs, he’s still thinking about the magic of materials. “If you go out on a golf course with a club whose face has the properties of our steel, there are good technical reasons for it to have a lot better spring. But how much more is the club worth if the metal is meteoric iron?” Olson points to a report in the August 25, 2000, issue of Science that suggests that the isotopes of a meteorite will tell you whether it comes from outside the solar system – which means that you could be whacking your Strata Tour Professional to the green with a club whose business end hurtled to Earth from beyond Pluto.
“That’s powerful stuff,” says Olson. “If you knew you had that mysticism in your golf club, what would it be worth? I’d like to know.”
***
Most people, and certainly most scientists, think that medieval alchemists like Nicholas Flamel were deluded cranks obsessed with the goofy idea that you could transmute lead and other chemical elements into gold. But Olson thinks that’s a misinterpretation. “The alchemists weren’t protochemists,” he says. “They were materials scientists. They realized that the whole point was to transform raw matter into something of value.” And value didn’t come only from economy or technological improvements; it had to do with art, even mysticism. “These guys were striving for a unification of the spiritual and material worlds.”
As an educator, Olson wants to do his part in re-unifying these worlds. To that end, he helped create the Engineering, Design, and Communication course for incoming engineers. Involving English teachers and science profs, the course attempts to create a “techmanities” curriculum that brings C. P. Snow’s famous two cultures – science and the humanities – together. “Today, I don’t think either liberal arts or a technical education has got what it takes,” says Olson. “The answer is somehow a fusion of the two, which I suppose is the new alchemy.” Organized into teams and assigned real-world problems, budding engineers interact with clients, survey markets, and design Web sites.
“It wasn’t a normal class,” says Brennan O’Donnell, a spiky-haired blond who took the course a few years ago. “We were only freshmen, and we were dealing with clients. It was very hands-on. A lot of people hated it. They came in book smart but didn’t want to do real-world work.”
In 1998, Olson decided to come up with the ultimate techmanities design project, one that would fuse materials science with history, literature, and myth. He also wanted to do something nifty with the Ferrium C69 his company wanted to sell. “The sword runs deep for everybody,” says Olson. By focusing on a Dragonslayer, Olson not only emphasized the mythology of the material, but could tiptoe around the ungodly amounts of carnage wreaked by these human meat cleavers. “There’s a dark side of the sword, and we wanted to avoid that. That’s why we put it in the context of man versus supernatural evil.”
And thus was born an academic project that, as one of Olson’s earliest teams described it in its final paper, represented “a bizarre combination of science and science fiction.” Megan Frary was on that team, one of about seven groups that have researched different aspects of the project over the last few years. “Most classes are just problem sets,” she says. “This was different.”
While a squad of upperclassmen in Olson’s materials design course researched the chemical composition of the blade, Frary’s freshman team surveyed the sword market. They spoke to many collectors, but they didn’t get much response from bladesmiths, a subculture of craftspeople who have reconstructed the almost dead art of hand-forging knives and swords. These folks sell a lot of weapons to members of medieval reenactment groups like the Society for Creative Anachronism, but most care too much about the historical accuracy of their art to make “fantasy swords” for D&D geeks who want replicas of their +2 Demon Banes. Most bladesmiths probably deleted the email from Frary’s team the second they saw the word dragon.
One bladesmith who eventually contacted Olson was Richard Furrer, a young, highly accomplished blacksmith who works out of his garage in Sturgeon Bay, Wisconsin. Furrer picked up blacksmithing as a hobby when he was a history of technology major at the University of Wisconsin-Madison. His first swords were Japanese katana blades, but these days he’s drawn to the delicate patterning and elegant lines of Indian and Persian court swords. He is also one of only a handful of people on the planet who know how to make wootz steel – a strong ancient steel first created in crucibles in central India and later used in the legendary Damascus swords of the Saracen warriors.
As a metallurgy buff and bladesmith, Furrer was attracted to a project that married avant-garde steel with the ancient labor of the forge. “Smelting was high technology 2,000 years ago,” he says. “It’s an incredible technological leap to take a colored rock and make a functional piece of art from that. What intrigues me about Dragonslayer is that they’re essentially doing the same thing, but starting with the materials of today. Developing C69 on computers is extremely cutting-edge – if you’ll pardon the pun.”
Furrer’s email arrived at a fortuitous moment: Olson needed the seax for the Materials Solution show, and Furrer had a slot open in his schedule. In short order, QuesTek sent him a 7-inch-long, 3-inch-diameter, 7-pound cylinder of Ferrium C69 with four holes bored through its length. Furrer sliced up this rather ungainly package and set to work. He bought the oosic piece from a fossil and mineral supplier for $100. A couple of weeks later, Furrer delivered Beowulf’s blade.
After the Materials Solutions show closed, Furrer trekked from Sturgeon Bay to Evanston to meet Olson in the flesh. A thickset 30-year-old with shoulder-length hair, a black beard, and a silver serpentine armband, Furrer looks even more like a blacksmith than Olson looks like an engineering prof. Shortly after walking into the QuesTek offices, Furrer handed Olson a gift: a 390-gram chunk of iron meteorite in a plastic baggie. (Similar material goes for about $50 a pound over the Net.) Furrer’s sample came from the Nandan meteorite that fell in China around the end of the 15th century, and it looked like an evil hunk of barbarian ordnance that had been corroding at the bottom of the sea for a thousand years. “That’s some real nasty material,” said Furrer.
When it comes time to make the actual Dragonslayer, similar chunks of space rock will have to be purified in order to furnish the iron for Ferrium C69. For this task, Olson has enticed Harvey Abramowitz, a metallurgist and a professor of mechanical engineering at Purdue University Calumet. Abramowitz will start by dissolving the meteorite in an aqueous electrolytic solution. Pure iron will precipitate out of the solution onto an electrode, leaving the impurities – like nickel, copper, and gallium – behind. The iron will then be sent to Allvac, a steel manufacturer in North Carolina; with carbon infused in the form of graphite powder, QuesTek’s recipe will become Ferrium C69. Then 5 pounds or so will be sent to the Dragonslayer bladesmith. Olson hasn’t yet decided who the lucky artisan will be, but he would like to maximize the mystique of the weapon by enlisting a well-known master, who can earn as much as $7,000 per dagger.
Furrer is interested, but he hasn’t spent much time working with rich alloys like Ferrium C69. In fact, no one has. “I’ve forged a lot of different steels and non-iron-based materials, but this stuff is unique.” Bladesmiths steer by color: As the material heats up, it passes from dull red to brightening orange, and somewhere along the way it reaches the claylike consistency that allows them to hammer it to shape. In order to protect the metal’s internal structure, Furrer had to bring C69 to a very high temperature, a bright orange verging on white. As soon as he started to pound the metal, the material hardened in the air, making it tougher to work. Still, the seax turned out fine. When Furrer tried to weld C69 with another steel to create a pattern-welded blade, however, he ran into trouble. For a good weld to hold, the atoms in the two materials must form covalent bonds, but the chromium in the C69 played hard to get. After 20 hours, stresses warped the edge of the welded blade beyond repair. “It died a horrible death,” says Furrer. “Rebar.”
Another expert who’s intrigued is Kevin Cashen, a friend of Furrer and a master bladesmith from Michigan whose speciality is pattern-welded swords from the early Middle Ages. “I like the idea of a nearly 2,000-year-old technology talking in the same room as computers,” says Cashen. He especially likes the idea of slicing through a katana blade. “There’s a lot of hype in Western culture’s fascination with Japanese swords,” he says with slight exasperation. “People will believe anything. So to make this Western sword out of a fancy alloy, and then cut a Japanese blade – it’s like sweet justice.”
Though bladesmiths are knocking on Olson’s door now, one gets the distinct sense that he wants to squeeze more juice out of the project before it’s completed. He wants to bring more students into the design process, and to hook up with other university departments, even seminaries. “In a way, it will be disappointing when it ends,” he admits. “I want the story to keep going.”
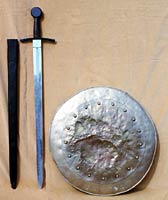